Projects
Here we list the current projects we are pursuing. Our future projects will expand in areas in which our industrial partners are interested.
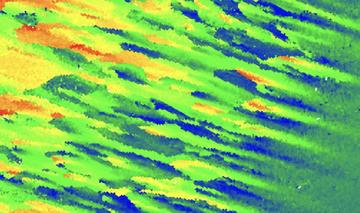
NMR and MRI are techniques that use the interactions of atoms with external magnetic fields to look inside materials, objects and organisms to study their composition (NMR) and provide images (MRI). They are used very widely in scientific research, medical research and in industry and medicine. Put simply, the stronger the magnetic field available the better these techniques work. Unfortunately, obtaining large magnetic fields (typically 20 -30 times strong than a fridge magnet) generally requires expensive magnets, which are usually wound from long lengths of superconducting wire. It would be ideal to be able to produce these very large magnetic fields in a much simpler fashion to provide convenient and cheap desktop systems. Making these systems widely available and cheaper would allow more scientists, engineers and medical researchers to have access to this equipment, and to use it more often. The importance of this proposed project is underlined by the active participation and practical help offered by our three industrial partners.
We are proposing to use ceramic bulk (in disc- or ring-form) superconductors, rather than complex solenoidal coils made from superconducting wire. The three main challenges that must be overcome to do achieve this are (i) making bulk superconductors of sufficient size and uniformity, (ii) making the magnetic field they produce highly uniform, and (iii) developing a practical way of charging bulks samples with magnetic field. To address the first two challenges the Cambridge group, with extensive experience of the fabrication and manufacture of these bulk superconductors, is going to partner with the Oxford group, who have experience of using advanced microscopy to look carefully at the fine details of the manufacturing process. To magnetise the bulk superconductors, we propose to discharge, over a period of several milliseconds, the energy stored in a bank of capacitors into a conventional coil magnet made of copper. Such a copper coil would overheat and melt if were to generate a large magnetic field continuously. However, using this pulsed field magnetisation technique, we can achieve the required field over a short period of time, but long enough to allow the bulk superconductor to "capture" the magnetic field.
We will consider the project successful if we can replace the conventional, permanent magnet of an existing NMR system, provided by our industrial partner, with our prototype bulk superconductor based system and demonstrate that it operates effectively at the proton resonance frequency of 200 MHz, rather than at 90 MHz, which is typical of existing permanent magnet systems and a limiting feature of this technology.
Project funded by EPSRC (EP/T014679/1)
Project partners
- Prof David Cardwell, Dr John Durrell, Engineering Department, University of Cambridge
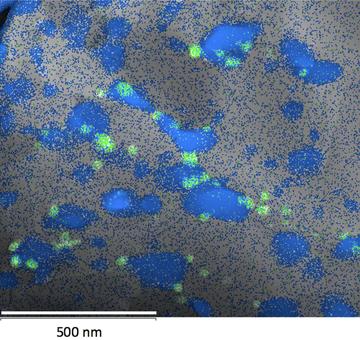
Bulk superconductors are dense pellets of superconducting material that can be used as compact permanent magnets. Harnessing the ability of these materials to produce considerably higher magnetic fields than conventional ferromagnets will be transformative for a wide range of devices for biomedical and energy applications. These materials have the added safety benefit that the magnetic field can be switched off. However, their enormous potential has yet to be realised in commercial devices for a number of reasons. High temperature superconducting bulk materials, such as YBCO, have the ability to produce very high fields, but they are expensive to produce, cannot be made with large diameter and suffer from relatively poor sample-to-sample reproducibility. Lower temperature superconductors like magnesium diboride (MgB2) are much cheaper and easier to process, but they require expensive and bulky cooling systems. Furthermore, all bulk samples present the additional challenge that they initially need to be magnetised using an external field.
This project involves designing and building a desktop sized magnet to demonstrate that these challenges can be overcome in practical devices by integrating state-of-the-art cryogenics and pulsed magnetisation systems with high performance, low-cost MgB2 superconductor. A bespoke cryostat will be developed by our team at the Rutherford Appleton Laboratory, who are experts in compact and efficient cryocoolers for space applications and coils will be incorporated into the cryostat, enabling the bulk superconductor to be magnetised in situ. The nano-scale structure of the MgB2 material will be optimised for operation at higher temperatures using a novel powder processing strategy, and large, high density samples will be manufactured using the commercial diamond presses at Element Six.
Preliminary experiments will be carried out using the demonstrator magnet, to assess the feasibility of using this technology for magnetically targeted drug delivery, with collaborators at the Institute of Biomedical Engineering in Oxford. More complex shapes of superconductor will be explored, with a view towards developing more sophisticated devices for selected applications such as MRI in future projects.
Project funded by EPSRC (EP/P026427/1)
Project partners
- Dr John Durrell and Prof David Cardwell, Engineering Department, University of Cambridge
- Dr Matt Hills, Dr Tom Bradshaw, Cryogenics and Magnetics Group, Rutherford Appleton Laboratories
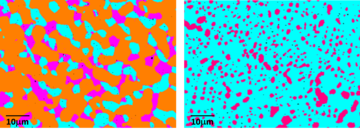
A key technology in the manufacture of high field magnets is the creation of high quality superconducting joints between individual lengths of superconducting wire. In the future it is likely that magnet designers will require these joints to be formed between dissimilar superconductors, including between low temperature superconducting (LTS) and high-temperature (HTS) materials. This project aims to develop and demonstrate the performance of new Pb-free superconducting solder formulations for LTS joints and also to develop and test joining methods for NbTi and Nb3Sn wires to other superconductors, including MgB2 and HTS conductors. We will use advanced analytical microscopies, testing of fatigue performance and superconducting property measurements to relate the structure of joints to their engineering performance. The image shows EDX chemical phase maps of In-Sn-Bi superconducting solders of two different alloy compositions.
Current projects
- Pb-free superconducting solders
- Industrial partner: SMT
- CfAS researcher: Tayebeh Mousavi (previously supported by EPSRC IAA funding)
- Solder-free joints
- Industrial partner: Cryox
- CfAS researcher: Greg Brittles (supported by EPSRC DTA studentship)
- Persistent mode superconducting joints
- Industrial partner: SMT
- CfAS researcher: Tim Davies (supported by EPSRC Industrial CASE studentship) [/collapse]
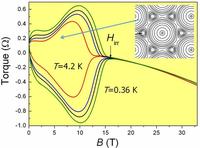
A challenge in engineering superconducting materials is to understand how to enlarge the critical surface defined by critical temperature Tc, upper critical magnetic field Bc and critical current densities Jc. Many of the newly developed superconducting materials have extremely high values of critical field and to study them one need a field larger than can be generated using a superconducting magnet. We will use a series of superconducting magnets up to 21 T in a small bore and up to 17 T in a large bore. Oxford hosts the Nicholas Kurti high-magnetic field facility, a pulsed system that provides the highest magnetic fields available in the UK. It is powered by a 7 kV capacitor bank and has been capable of generating fields of up to 45 T, but is currently being upgraded to a 15 kV system (funded by an EPSRC grant) aimed to increase the maximum field to 70 T. We will design and build test apparatus for measuring superconducting critical currents as function of temperature and magnetic field. These studies will be extended to include measurement under strain as well as optical strain gauges in extreme conditions. Studies odf superconducting properties at microscale will focus on transport measurements, torque magnetometry, nanocalorimetry, tunnel diode oscillators. We will continue to develop the experimental tools to perform measurements in ultra high magnetic fields in Oxford and plan a programme of measurements to characterise the high-field physics of new superconductors discovered in Oxford and elsewhere.
The best low noise microwave amplifiers currently available are based on high quality high electron mobility transistors (HEMTs). However, even the best HEMT devices reach noise temperatures at least an order of magnitude away from the ultimate quantum limit. To reach this limit, the new technology of superconducting parametric amplifiers is emerging. Unlike the inherently dissipative transistor-based amplifier, a parametric amplifier relies purely on non-linear reactance to convert energy between different frequencies in a mixing process. Superconducting devices are ideally suited to this application, since a strong non-linearity can be easily realised in an intrinsically dissipation-free device. To reach high gain, the signal must interact strongly with the non-linearity and this can be realised by making a device with a very long propagation length – a travelling wave parametric amplifier (TWPA) – likely to be promising for commercial devices due to much larger bandwidth and higher dynamic range than recently developed resonator-based approaches. This project area will explore both kinetic inductance and Josephson junction designs for TWPAs and their application in quantum technology and astronomical receivers, with the goal of integrating the best TWPA designs into quantum test probes.
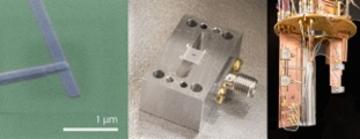
Many of the most exciting advances in quantum technologies rely on resonant circuits fabricated in superconducting materials. Al or Nb films can be used for these circuits, but many of the most impressive results have been achieved with nitride films like TiN as the superconducting material. In Phase 1 of the centre we will establish a flexible materials discovery facility to grow and test a wide range of thin film materials for application in this area. The programme will have two stages;
- Materials deposition and testing to determine promising candidates
- Optimising thin films for testing in real circuit designs
New techniques in flux line imaging and mapping allow us to explore the way that supercurrents interact with cable and filament geometries and with the local microstructures. This will allow us to study the mechanisms by which quenches initiate and the flux flow mechanisms in multi-filamentary conductors.
In Phase 1 of the centre, we will design and build test apparatus for:
- magneto-optic mapping of conductors under medium fields and carrying currents up to Jc values to initiate local quenching, and
- high resolution scanning magnetometry will enable direct studies of flux pinning in inhomogeneous materials to inform the development of microstructures with optimised properties